Science
Related: About this forumQuantifying the Photolytic Fate of Fluorinated Pharmaceuticals and Pesticides.
Last edited Sun Feb 25, 2024, 08:43 AM - Edit history (1)
The paper I'll discuss in this post is this one: Fluorinated Pharmaceutical and Pesticide Photolysis: Investigating Reactivity and Identifying Fluorinated Products by Combining Computational Chemistry, 19F NMR, and Mass Spectrometry Akash P. Bhat, William C. K. Pomerantz, and William A. Arnold Environmental Science & Technology 2024 58 (7), 3437-3448
The extreme stability of the carbon fluorine bond has made it persistent in the environment, and has resulted in the serious pollution of all terrestrial matrices on this planet, including but not limited to biological matrices. Garnering most attention are the compounds related to consumer and industrial products such as Teflon, certain upholstery protection products, lubricants, coatings, and fire fighting foaming agents, collectively know as "PFAS." One of the concerns connected with the toxicology of the compounds concerns the fact that fluorine in organic molecules has properties that mimic hydroxyl functions, -OH groups, that are very common in all of the major biologically active classes of molecules, notably sugars, including those involved with DNA and RNA, proteins, in particular those rich in the residues serine, threonine and tyrosine, and even certain important lipids signaling, sphingosines, for one example. Fluorine, like oxygen, can form hydrogen bonds, and thus can interfere with biological processes. In addition, trifluoromethyl and difluoromethyl groups are electron withdrawing, and thus can play a role in mimicking the ubitiquous carboxylic acids in living systems. Combined with their stability, which can vastly increase their resistance to metabolism, fluorocarbon moieties are often used by agrochemists and medicinal chemists to lengthen the lifetime and activity of pesticides and medications. Because these compounds are either deliberately sprayed into the environment in the case of pesticides, or excreted in urine in the case of medications, either intact or partially metabolized, and thus find their way into rivers, streams and other bodies of water in the outfall pipes of sewage treatment plants, or groundwater from septic systems, they can persist for a very long time, adding to the PFAS problem, in particular because these molecules are designed for biological activity.
The authors here focus on the mechanism of degradation of fluorinated compounds using radiation, and explore analytical chemistry techniques in following degradation products.
From the introduction to the paper:
The most common method used for identification of fluorinated degradation products of pesticides and pharmaceuticals is liquid chromatography with nontargeted high resolution mass spectrometry (LC-HRMS). (17) It was reported that using only LC-HRMS to quantify fluorinated compounds can result in fluorine mass balances falling short, with up to 90% of fluorine being unaccounted for. (17?20) Recent studies on fluorinated product formation and identification during photolysis and advanced treatment processes have reported more complete product identification by complementing LC-HRMS with quantitative 19,F-nuclear magnetic resonance spectroscopy (NMR) analysis. (9?11,21,22) An NMR spectrum contains all fluorinated product peaks, and the chemical shifts provide insight into the identity of the functional group and its chemical environment. With the broad range of fluorine NMR shifts (?400 ppm) and the 100% abundance of 199F isotope of fluorine in the environment, 19F NMR has been reported to be a highly suitable method to detect fluorinated compounds and products. (23) Also, by increasing the number of scans four times, the signal-to-noise ratio is doubled, allowing minor products to be detected. Using 19F NMR to quantify the products formed assists in evaluation of chemical structures identified using the semiquantitative (at best) LC-HRMS data. (10) For example, the photolysis of fluoxetine led to formation of norfluoxetine, TFA, and fluoride. (10) On the other hand, the 19F NMR spectra after photolysis of sulfoxaflor showed that all product peaks formed were similar in shift to the parent peak, indicating that all products were closely related to each other and the parent structurally, making evaluation of product data obtained via LC-HRMS simpler, faster, and more precise. (9) In cases such as this, however, where multiple 19F NMR peaks are within several ppm the parent peak, it is difficult to assign these NMR peaks to the specific structures identified by LC-HRMS to have modifications remote to the fluorinated functional group of interest, which hinders quantifying the relative amounts of different products. (24)
The 19F NMR shifts for common products like fluoride (F), trifluoroacetate (TFA), and difluoroacetate (DFA) are known and are easily identified and quantified. For new fluorinated product structures formed via degradation of pesticides and pharmaceuticals, it is difficult to predict the upfield or downfield movement of 19F NMR shifts with respect to the parent compounds, thereby potentially making accurate matches of products identified using LC-HRMS with the NMR data challenging and complicated. Major products may go unidentified, unless these products are matched to their 19F NMR shifts. With the unavailability of mass-labeled standards for quantitative mass spectrometry for these newly identified fluorinated products and the difficulty in separating products using preparative scale chromatography, computational calculations for 19F NMR shifts could pave the way for precise and accurate identification of products leading to matches between experimental 19F NMR spectra and LC-HRMS product structures.
Computational methods can be used to obtain 19F NMR shifts for fluorinated organic compounds, even in the absence of standards...
The synthetic difficulty of making PFAS standards is, by the way, profound, and 19F NMR as the authors note can overcome these problems, albeit at the expense of sensitivity.
A graphic in the article gives examples of commercial compounds which feature fluorinated carbons:
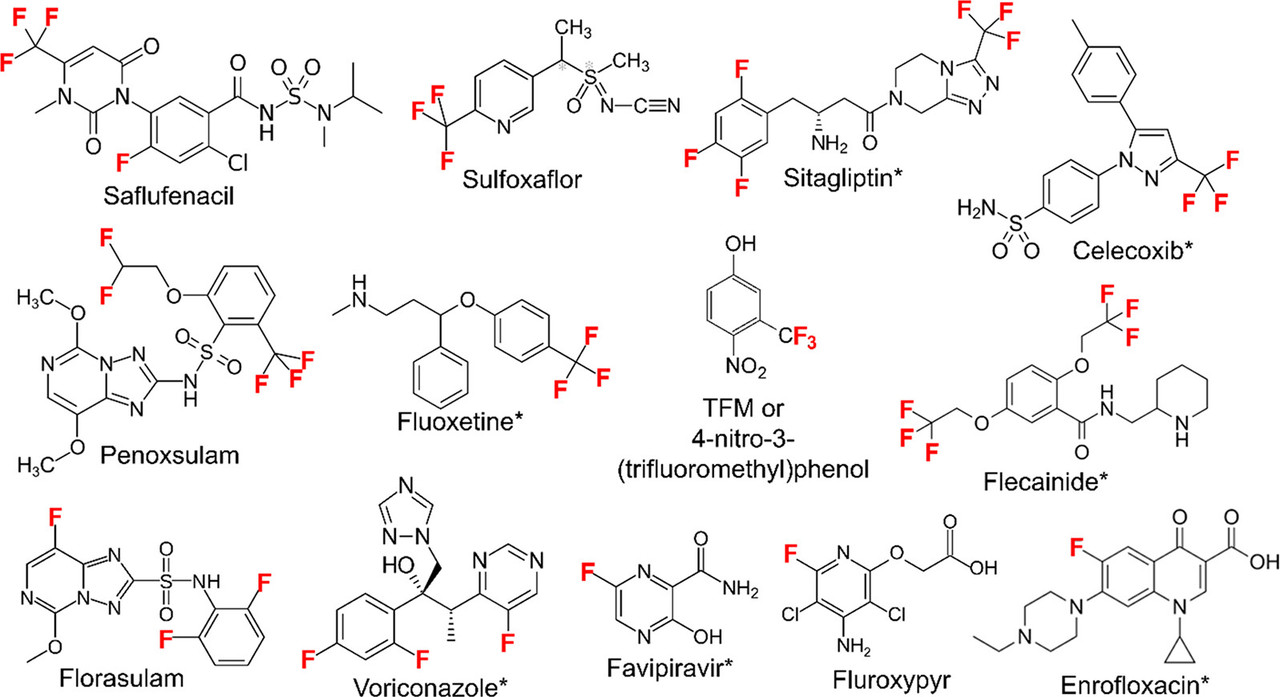
The caption:
The radiation used by the authors to study the degradation are as follows: 255, 275, 308, 365, and 405 nm, provided by LEDs and mercury lamps. All of these wavelengths, except the visible violet 405 nm are in the UV range as opposed to the visual range. Our wonderful industrial and agricultural efforts to destroy the ozone layer means that we can be assured of the degradation of these molecules over the long run, albeit at the expense of limiting or completely destroying life on Earth. (Problem solved?)
The results of the experiments and the products are shown graphically in the rather lengthy supplemental information, and happily in some cases, one of the products is the fluoride ion but other fluorinated species, notably the problematic TFA, trifluoroacetic acid, is well represented. Shorter wavelengths, unsurprisingly, are more efficient in general in producing the fluoride ion it seems.
An interesting paper.
Have a nice weekend.

lastlib
(23,244 posts)How does it affect flouride-propyl-people ethers?
(see what I did there? )
eppur_se_muova
(36,268 posts)I'm guessing they have specialized functions, like ecdysone inhibitors or promoters that *hopefully* have minimal effects on other species.
NNadir
(33,525 posts)Activity is the biggest issue, as it is in medicinal chemistry, but closely followed by specificity.
I briefly worked in the agrochemical field, primarily with respect to (gasp) genetic modification, but I was familiar with residue testing, which is basically connected to metabolism.
In my opinion the most difficult environmental problem to solve after climate change is the effects of agriculture. I would rate the problem of fertilizer - particularly with respect the generation of ozone depleting and GHG driving N2O - and of course, phosphorous, somewhat higher than pesticide use.
We're in a hell of a spot with 8 billion mouths to feed.